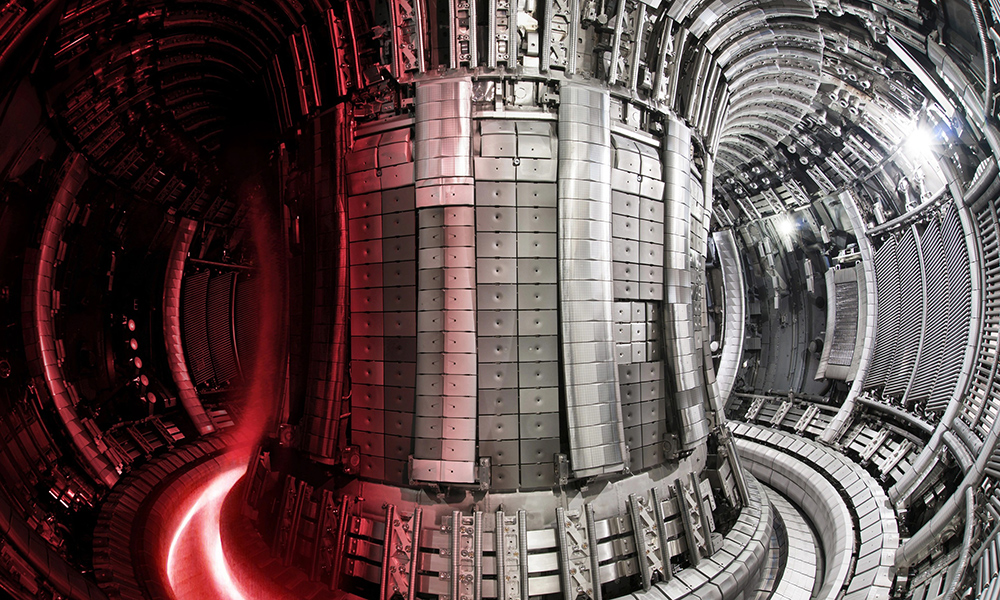
在众多有希望的净零解决方案中,核聚变最突出的优势在于其规模及雄心。太阳的能量也来自核聚变反应,如果能够成功复制这一过程,用斯蒂芬·霍金的话说,人类就可以解锁“取之不尽、用之不竭的能源供应,同时既不会造成污染,也不会使全球变暖。”然而,几十年来,核聚变领域进展缓慢,哪怕有一些突破,也是时断时续。
这种情况可能即将改变。得益于广泛的国际合作及数以亿计的公私投资,科学家们最近在核聚变反应的持续时间和功率输出方面取得了一系列有意义的进展。
传统核电站的能量源自核裂变,即原子发生分裂,核聚变指的是一对轻原子核结合形成一个较重的原子核。核聚变发生时,大量能量得到释放:相当于裂变的四倍、燃烧化石燃料的近400万倍。从安全性上看,核熔毁事故几乎不可能发生,而且只会产生少量寿命相当短暂的放射性废物。
在中国、俄罗斯、美国和几个欧洲国家政府的支持下,一个由全球物理学家组成的联盟正在努力将核聚变不可思议的前景变成现实。由于得到了源源不断的资金注入,科学家们最近取得了一系列重大进展,解决了若干科学难题——尤其是触发核聚变反应所需的超高温度。在太阳中心,原子的结合发生在大约1,000万摄氏度左右;地球引力要小得多,因此需要的热量至少是太阳上的10倍。
由于目前没有任何材料能够承受如此高温,科学家们设计了不同的方法来约束超高温等离子体(发生聚变的带电粒子云),以确保能量持续输出。位于美国加利福尼亚州的国家点火装置(National Ignition Facility)正在研发如何利用高功率激光将聚变燃料压缩到微小空间中,而世界其他地区的研究人员则倾向于通过强磁场来约束聚变燃料。
充满希望的进展
欧洲联合环(Joint European Torus)是后一个研究领域的先驱。位于英国的欧洲联合环实验室最近打破了自己的核聚变能量世界纪录,成功输出了相对较高的热能水平并保持了5秒。其中输出的能量略低于60兆焦耳——只够烧开几十个水壶——但它标志着在追寻可持续核聚变能量的道路上迈出了重要一步。
“5秒的脉冲和59焦耳的能量可能听起来不多,但这表明我们有能力实现持续放电,创造高核聚变产额。”欧洲联合环研究团队的联合负责人、核物理学家若埃勒·马尤说。“我们现在画出了一张蓝图,可以在未来扩大作业规模,争取大幅提升输出时间。”
然而,全球核聚变研究仍然面临巨大障碍。欧洲联合环上述打破纪录的实验消耗的能量比产生的能量大得多——目前全球任何团队都未能成功从核聚变中获得净能量——而用来控制等离子体的磁铁升温过快,无法长时间操作。
尽管如此,研究还是取得了一些进展。今年早些时候,中国科学家成功实现了一次维持17分钟的核聚变反应,尽管该实验使用的燃料不适合用于大规模发电。然后是国际热核聚变实验堆(International Thermonuclear Experimental Reactor)计划,这是全球最雄心勃勃的核聚变项目,如果一切顺利,将在本世纪20年代中期投入使用。
位于法国南部的国际热核聚变实验堆的设施正在建设中,借鉴即将退役的欧洲联合环项目的数据,规模比后者要大得多,它使用的是能够承受更高温度的材料,理论上可以让核聚变实验持续足够长时间,从而实现输出的能量大于消耗。但专家们表示,这在本世纪40年代末之前都不太可能实现,等它成功了,也不确定何时才能够提高聚变能量的成本效益。
“雨后春笋般的初创企业”
另一个严峻的问题与氢的两种同位素——氘和氚有关,需要它们为国际热核聚变实验堆的核聚变反应提供燃料。氘可以从海水中大量提取,但氚则异常罕见(据信全世界只存在20千克氚)。为了克服这个问题,人们正在探索在核聚变过程中“繁殖”氚的技术,马尤说,这项技术可能需要几十年才能够实现。
私营企业进场了。近年来,在数以亿计的风投资金的推动下,涌现了一批寻求替代性解决方案的初创公司。其中包括谷歌(Google)和雪佛龙(Chevron)支持的TAE Technologies公司,这家公司位于美国加州,正在研发无氚聚变反应堆。
TAE的首席执行官米希尔·宾德鲍尔认为,通过用非放射性的氢-硼替代稀缺的氢同位素氚,他的团队可以规避燃料可用性问题,最快在本世纪30年代初就能够实现商业能源生产。
“我们的机器比其他核聚变反应堆要小得多;一台机器可以提供一个小城市的电力,大约是两辆双层巴士的大小。”宾德鲍尔说,“这意味着它们更容易集中生产,释放规模经济效应。”
TAE对成本的前景也很乐观——宾德鲍尔称,一开始每千瓦时的成本差不多会处于中间位置,介于核裂变的高价和天然气的低价之间。随着生产复杂零部件的成本下降,能源成本也会下降。
然而,全球科学界并非所有人都如此自信。位于澳大利亚的格里菲斯大学(Griffith University)的绿色能源专家、名誉教授伊恩·洛在20世纪60年代还是一名物理学本科生,那时他第一次听说核聚变能源的商业化,当时的预测是至少还需要50年。半个多世纪过去了,他担心还需要50年。
“是的,已经有了令人激动的进展,但归根结底,目前每一个有希望的核聚变反应堆都仍然处于研究阶段,而我们现在就需要清洁能源解决方案。”洛说,“我们已经拥有了能够生产高性价比零碳电力的可再生能源,扩大这类能源的规模才应该是当下的重点。”(财富中文网)
译者:Agatha
在众多有希望的净零解决方案中,核聚变最突出的优势在于其规模及雄心。太阳的能量也来自核聚变反应,如果能够成功复制这一过程,用斯蒂芬·霍金的话说,人类就可以解锁“取之不尽、用之不竭的能源供应,同时既不会造成污染,也不会使全球变暖。”然而,几十年来,核聚变领域进展缓慢,哪怕有一些突破,也是时断时续。
这种情况可能即将改变。得益于广泛的国际合作及数以亿计的公私投资,科学家们最近在核聚变反应的持续时间和功率输出方面取得了一系列有意义的进展。
传统核电站的能量源自核裂变,即原子发生分裂,核聚变指的是一对轻原子核结合形成一个较重的原子核。核聚变发生时,大量能量得到释放:相当于裂变的四倍、燃烧化石燃料的近400万倍。从安全性上看,核熔毁事故几乎不可能发生,而且只会产生少量寿命相当短暂的放射性废物。
在中国、俄罗斯、美国和几个欧洲国家政府的支持下,一个由全球物理学家组成的联盟正在努力将核聚变不可思议的前景变成现实。由于得到了源源不断的资金注入,科学家们最近取得了一系列重大进展,解决了若干科学难题——尤其是触发核聚变反应所需的超高温度。在太阳中心,原子的结合发生在大约1,000万摄氏度左右;地球引力要小得多,因此需要的热量至少是太阳上的10倍。
由于目前没有任何材料能够承受如此高温,科学家们设计了不同的方法来约束超高温等离子体(发生聚变的带电粒子云),以确保能量持续输出。位于美国加利福尼亚州的国家点火装置(National Ignition Facility)正在研发如何利用高功率激光将聚变燃料压缩到微小空间中,而世界其他地区的研究人员则倾向于通过强磁场来约束聚变燃料。
充满希望的进展
欧洲联合环(Joint European Torus)是后一个研究领域的先驱。位于英国的欧洲联合环实验室最近打破了自己的核聚变能量世界纪录,成功输出了相对较高的热能水平并保持了5秒。其中输出的能量略低于60兆焦耳——只够烧开几十个水壶——但它标志着在追寻可持续核聚变能量的道路上迈出了重要一步。
“5秒的脉冲和59焦耳的能量可能听起来不多,但这表明我们有能力实现持续放电,创造高核聚变产额。”欧洲联合环研究团队的联合负责人、核物理学家若埃勒·马尤说。“我们现在画出了一张蓝图,可以在未来扩大作业规模,争取大幅提升输出时间。”
然而,全球核聚变研究仍然面临巨大障碍。欧洲联合环上述打破纪录的实验消耗的能量比产生的能量大得多——目前全球任何团队都未能成功从核聚变中获得净能量——而用来控制等离子体的磁铁升温过快,无法长时间操作。
尽管如此,研究还是取得了一些进展。今年早些时候,中国科学家成功实现了一次维持17分钟的核聚变反应,尽管该实验使用的燃料不适合用于大规模发电。然后是国际热核聚变实验堆(International Thermonuclear Experimental Reactor)计划,这是全球最雄心勃勃的核聚变项目,如果一切顺利,将在本世纪20年代中期投入使用。
位于法国南部的国际热核聚变实验堆的设施正在建设中,借鉴即将退役的欧洲联合环项目的数据,规模比后者要大得多,它使用的是能够承受更高温度的材料,理论上可以让核聚变实验持续足够长时间,从而实现输出的能量大于消耗。但专家们表示,这在本世纪40年代末之前都不太可能实现,等它成功了,也不确定何时才能够提高聚变能量的成本效益。
“雨后春笋般的初创企业”
另一个严峻的问题与氢的两种同位素——氘和氚有关,需要它们为国际热核聚变实验堆的核聚变反应提供燃料。氘可以从海水中大量提取,但氚则异常罕见(据信全世界只存在20千克氚)。为了克服这个问题,人们正在探索在核聚变过程中“繁殖”氚的技术,马尤说,这项技术可能需要几十年才能够实现。
私营企业进场了。近年来,在数以亿计的风投资金的推动下,涌现了一批寻求替代性解决方案的初创公司。其中包括谷歌(Google)和雪佛龙(Chevron)支持的TAE Technologies公司,这家公司位于美国加州,正在研发无氚聚变反应堆。
TAE的首席执行官米希尔·宾德鲍尔认为,通过用非放射性的氢-硼替代稀缺的氢同位素氚,他的团队可以规避燃料可用性问题,最快在本世纪30年代初就能够实现商业能源生产。
“我们的机器比其他核聚变反应堆要小得多;一台机器可以提供一个小城市的电力,大约是两辆双层巴士的大小。”宾德鲍尔说,“这意味着它们更容易集中生产,释放规模经济效应。”
TAE对成本的前景也很乐观——宾德鲍尔称,一开始每千瓦时的成本差不多会处于中间位置,介于核裂变的高价和天然气的低价之间。随着生产复杂零部件的成本下降,能源成本也会下降。
然而,全球科学界并非所有人都如此自信。位于澳大利亚的格里菲斯大学(Griffith University)的绿色能源专家、名誉教授伊恩·洛在20世纪60年代还是一名物理学本科生,那时他第一次听说核聚变能源的商业化,当时的预测是至少还需要50年。半个多世纪过去了,他担心还需要50年。
“是的,已经有了令人激动的进展,但归根结底,目前每一个有希望的核聚变反应堆都仍然处于研究阶段,而我们现在就需要清洁能源解决方案。”洛说,“我们已经拥有了能够生产高性价比零碳电力的可再生能源,扩大这类能源的规模才应该是当下的重点。”(财富中文网)
译者:Agatha
In the packed field of prospective net-zero solutions, nuclear fusion stands out in its scope and ambition. By successfully replicating the reaction that powers the sun, humanity could—in the words of Stephen Hawking—unlock an “inexhaustible supply of energy, without pollution or global warming.” Fusion development has progressed at a glacial pace for decades, however, and breakthroughs have been intermittent at best.
That could be about to change. With sweeping international collaboration and billions of dollars of public and private investment, scientists have recently made a series of meaningful advances in both the duration and power output possible from fusion reactions.
Whereas nuclear fission—the process that drives conventional nuclear power plants—involves the splitting of atoms, fusion takes place when a pair of light atomic nuclei combine to form a single heavier one. When this happens, an immense amount of energy is released: four times as much as fission, and nearly 4 million times greater than the burning of fossil fuels. From a safety standpoint, nuclear meltdown is practically impossible, and only a small volume of relatively short-lived radioactive waste is produced.
Fusion’s incredible promise is being pursued by a consortium of global physicists supported by China, Russia, the United States, and several European governments. With the funding taps open, significant progress has recently been made to overcome a litany of scientific challenges—not least the enormous temperatures required to trigger a fusion reaction. In the core of the sun, atoms combine at approximately 10 million degrees Celsius; on Earth, where gravitational forces are far, far smaller, at least 10 times as much heat is required.
As no known material can withstand contact with such scorching temperatures, scientists have devised different methods of confining super-hot plasma—a cloud of charged particles in which fusion occurs—to allow for continuous energy output. In California, the National Ignition Facility (NIF) is developing the use of high-powered lasers to compress fusion fuel into a tiny space, while researchers in other parts of the world favor confinement via strong magnetic fields.
Promising progress
The Joint European Torus (JET) is a pioneer of the latter. Defeating its own world record for fusion power, the U.K.-based JET laboratory recently managed to produce and maintain a comparatively high level of thermal energy over a five second period. A little less than 60 megajoules (MJ) of energy was generated—just enough to boil a few dozen kettles—but it marked a significant step forward in the quest for sustained fusion energy.
“A five-second pulse and 59 MJ of energy production might not sound like a lot, but it shows that we’re capable of achieving a sustained discharge that produces a high fusion yield,” says Joelle Mailloux, a nuclear physicist co-leading the JET research team. “We now have a blueprint for scaling up operations in the future, with the goal of maintaining output for much longer than a few seconds.”
Huge hurdles remain for global fusion research, however. JET’s record-breaking experiment used significantly more power than it produced—net energy gain from fusion is yet to be demonstrated anywhere—while the magnets used to contain the plasma warmed too quickly for extended operation.
Nonetheless, progress is being made. Earlier this year, Chinese scientists managed to sustain a 17-minute fusion reaction—albeit with a fuel source which isn’t viable for large-scale power production. Then there is the International Thermonuclear Experimental Reactor (ITER)—the world’s most ambitious fusion project which, all going well, will be operational by mid-decade.
Drawing on data from the soon-to-be decommissioned JET program, the far larger ITER facility in southern France is being constructed from materials that can withstand much higher temperatures, allowing, in theory, for fusion experiments to run long enough to produce more power than they consume. But that’s unlikely to happen before the late 2040s, experts say, and when it does, there’s no telling how quickly fusion energy will become cost-effective.
“A flurry of startups”
Another serious issue revolves around the two forms of hydrogen, deuterium and tritium, used to fuel ITER’s fusion reaction. Deuterium can be derived in abundance from seawater, but tritium is exceptionally rare (only 20 kilograms are thought to exist worldwide). To overcome this deficit, techniques for “breeding” tritium during fusion are being explored, Mailloux says, but again, that technology is likely decades from realization.
Enter private enterprise. A flurry of startups seeking alternate fusion solutions have emerged in recent years, propelled by billions of dollars of venture capital investment. Among those is Google- and Chevron-backed TAE Technologies, a California-based firm developing tritium-free fusion reactors.
By replacing the scarce tritium hydrogen isotope with nonradioactive hydrogen-boron, TAE chief executive Michl Binderbauer believes his team can sidestep problems around fuel availability and achieve commercial energy production as soon as the early 2030s.
“Our machines are far more compact than other fusion reactors; a small city can be powered by one roughly the size of a couple double-decker buses,” Binderbauer says. “This means they’ll be easier to centrally manufacture, unlocking economies of scale.”
When it comes to pricing, TAE is bullish—cost per kWh will start out mid-field, Binderbauer claims, somewhere between nuclear fission at the high end, and natural gas at the bottom. And as the cost of fabricating complex components comes down, so too will the price of energy.
Not all in the global science community are so confident, however. As a physics undergraduate in the 1960s, Emeritus Professor Ian Lowe—a green energy expert at Griffith University in Australia—first heard that commercial fusion power was at least 50 years away. More than a half century later, he’s worried that it still is.
“Yes, there have been exciting developments, but ultimately all would-be fusion reactors are still in the research stage, and we need clean energy solutions now,” Lowe says. “We already have renewables that can generate cost-efficient zero-carbon power; scaling those up needs to be our focus.”